Stimulation Artifact Cancellation
Live Stimulation Artifact Cancellation
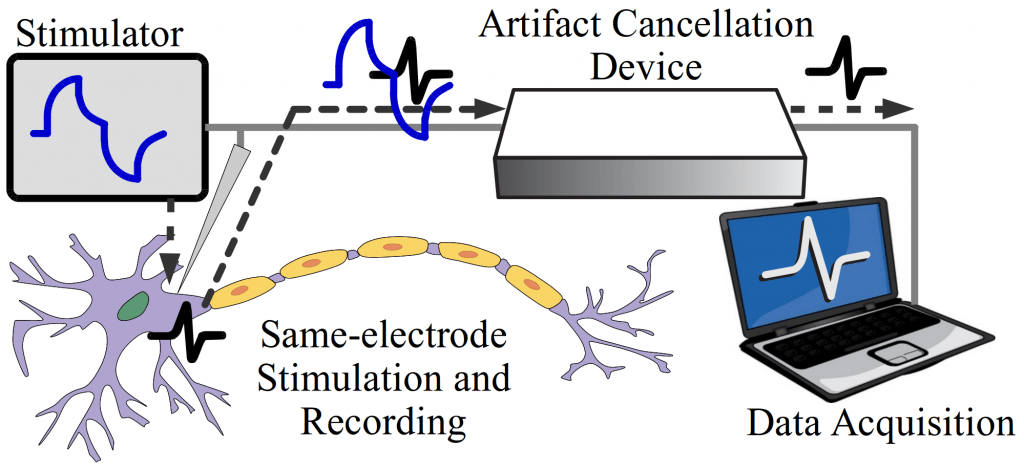
Publication
“A Hybrid Hardware and Software Approach for Cancelling Stimulus Artifacts During Same-electrode Neural Stimulation and Recording,” Stanislav Culaclii, Brian Kim, Yi-Kai Lo, and Wentai Liu, accepted by EMBC 2016.
Cross Frequency Coupling (CFC)
Cross Frequency Coupling (CFC) is the interaction between brain oscillations of different frequencies, and the coupling phenomenon has been observed in the brain of rodent and human. Phase-amplitude coupling (PAC) is a type of CFC, which describes the dependence between the phase of a low-frequency component and the amplitude of a high-frequency component of electrical brain activities. It has been claimed that the modulation of low frequency phase on high frequency amplitude plays a functional role in cognition and information processing, such as learning and memory. The change of PAC patterns has been associated with various neurological disorders, e.g., epilepsy and schizophrenia.
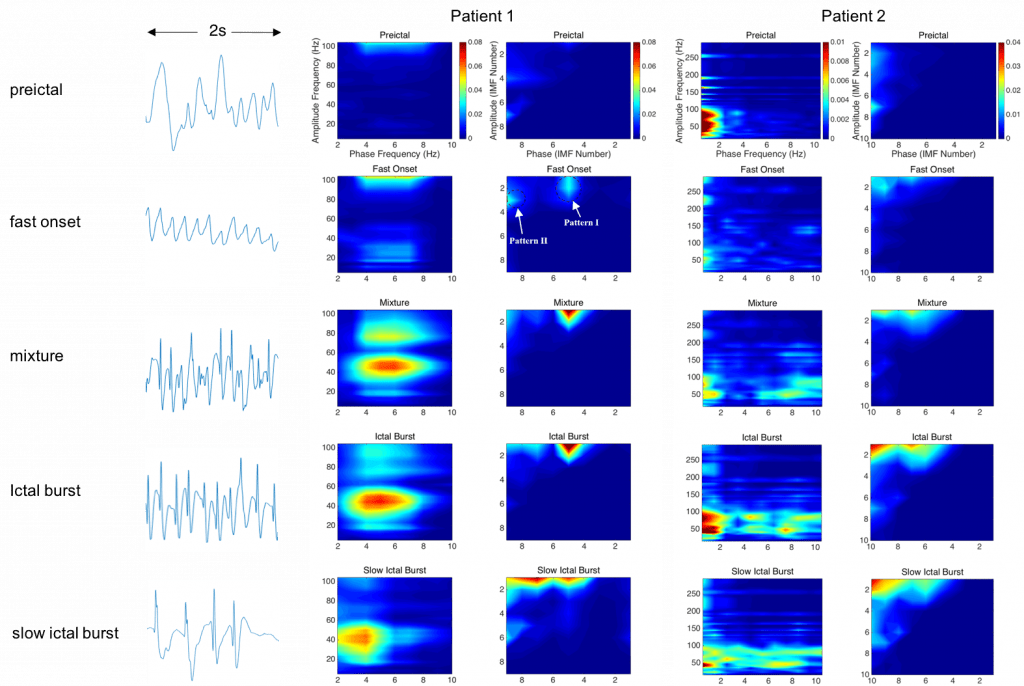
Non-stationary and nonlinear techniques for seizure detection algorithms
Epilepsy is one of the most common neurological diseases, affecting over 3 million people in U.S. and 50 million (~1%) people worldwide. An automated and accurate seizure detection method can be very helpful. Currently, most people use stationary and linear methods (i.e. Fast Fourier Transform (FFT), etc.) to analyze the signal. However, EEG signal is non-stationary and nonlinear in nature, thus these methods will introduce inaccuracy. Hence, we are developing non-stationary and nonlinear algorithms to improve the accuracy of seizure detection.
Collaborators
- Dr. Yue-Loong Hsin, Chung Shan Medical University, Taiwan
Electrode-based Bio-imaging
Our goal is to develop an Electrode-based Bio-imaging System that will be fMRI-competitive. Currently, fMRI is very popular since it offers high spatial resolution (~3mm); however, its temporal resolution is limited (~1s) and is impossible to be used in portable applications. EEG has excellent temporal resolution (~1ms), portable mobility that allowed to be used in daily life. Here, we devote to improving the spatial resolution of EEG in the aspects of both hardware and software.
1. Hardware
We developed a Focused Electrode that improves spatial resolution, recording SNR and reduce crosstalk and correlation with adjacent electrodes. The Focused Electrode is adaptive to the geometry parameters of electrode array including electrode size, pitch and source depth, such as EEG or ECoG. Simulation results show that the Focused Electrode increases the number of electrodes up to 7x in EEG and 30x in ECoG without overlapping information if the array covers half of head.
2. Software
We study the inverse imaging using realistic head model based on MR image (NFT toolbox). On one hand, we are studying the influence of various factors (i.e. noise, electrode number, head model, inverse solution, etc.) on inverse imaging; on the other hand, we are developing more accurate inverse imaging algorithms.
Collaborators
- Prof. Scott Makeig, SCCN, UCSD
- Dr. Yue-Loong Hsin, Chung Shan Medical University, Taiwan
Brain Dynamics and Source Localization
Brain imaging techniques can help us explore the function of human brain (i.e. memory, behavior, etc.) and help diagnosis and treatment of brain disorders (i.e. seizure, depression, etc.). Imaging tool with high temporal and spatial resolution is highly desirable. Currently, fMRI is very popular since it offers high spatial resolution (~3mm); however, its temporal resolution is limited (~1s) and is impossible to be used in portable applications. EEG has excellent temporal resolution (~1ms), portable mobility that allowed to be used in daily life. Our goal here is to improve the spatial resolution of EEG to be as good as that of fMRI.
We study the brain dynamics and source localization based on realistic head model (NFT toolbox). On one hand, we are studying the influence of various factors (i.e. noise, electrode number, head model, inverse solution, etc.) on the accuracy of inverse imaging; on the other hand, we are developing more accurate inverse imaging algorithms.
Collaborators
- Prof. Scott Makeig, SCCN, UCSD
- Dr. Yue-Loong Hsin, Chung Shan Medical University, Taiwan
Neural Signal Processing and Telemetry
Recording from a large number of neurons produces vast quantities of data that is highly difficult to extract and interpret due to noise and aggregation of multiple neural signals in a single recording site. In addition to conventional techniques like spike sorting, component analysis, de-noising detection, we are focusing on the cellular and molecular levels to understand the fundamentals of neural signals and behavior of large group of neurons. We found that the traditional deterministic channel models are insufficient for the description of the activities of real neurons. We are currently developing the stochastic kinetic modeling of sodium channel and its validation with the measurement of gate current due to transmembrane protein movements in the order of several picoamps with the bandwidth of several hundred of MHz.
Selected Publications
- “A 12-channel 6mW Wireless Neural Recording IC with On-the-fly Spike Sorting and UWB Transmitter,” M. Chae, W. Liu, Z. Yang, T. Chen, J. Kim, M. Sivaprakasam, and M. R. Yuce, To appear in International Solid-State Circuits Conference, February 2008.
- “An Integrated Multi-Channel Neural Recording System,” M. Chae, W. Liu, and M. Sivaprakasam, BMES Annual Fall Meeting, September 2007.
Collaborators
- Huntington Medical Research Institutes
- Arizona State University
Non-Invasive Functional Magnetic Stimulation
Neural stimulation is commonly accomplished by a voltage or current pulse through a microelectrode. Ideally, a method would exist which inherently had zero net charge transfer, required only simple driver circuitry and was completely isolated from the tissue to reduce circuit failure due to corrosion and fouling by protein deposition. Magnetic stimulation achieves these goals. The presence of scar tissue or deposited proteins is irrelevant because the magnetic permeability of tissue is near that of free space. Excitation arises from the magnetic field which generates a current across the membrane of the cell which changes the resting potential of the neuron and triggers an action potential (the fundamental signal generation mechanism neurons employ). We are currently studying the fundamental mechanisms of magnetic stimulation, developing models and verifying through in vitro experiments.
Selected Publications
- “Functional Magnetic Stimulation for Epiretinal Prosthesis”, E. Basham, W. Liu, and M. Sivaprakasam, Abstract B254, Association of Research in Vision and Ophthalmology Annual Meeting, May 2005.
Collaborators
- Santa Clara University